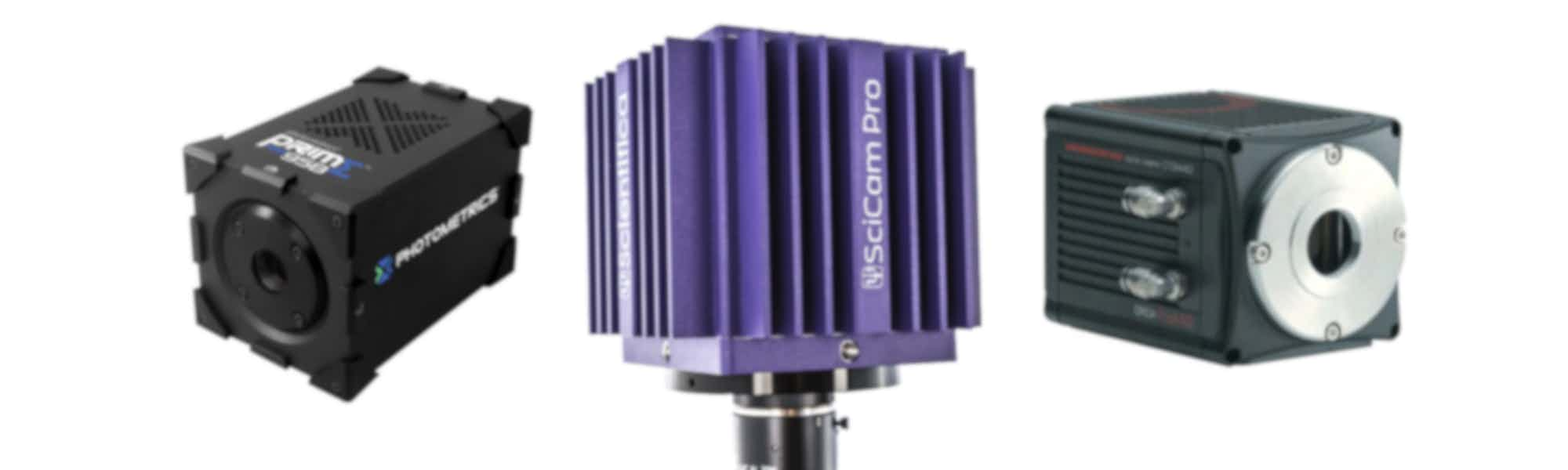
Cameras for fluorescence imaging: A comparative guide
In order to detect the often low levels of fluorescence emitted by samples, cameras used in fluorescence microscopy need to have a high sensitivity and low noise, enabling them to capture as many photons emitted from the sample as possible, with minimal noise.
Monochrome cameras are usually more suited to fluorescence imaging. They do not have a colour filter array, allowing more photons to reach the photosensitive sensor, increasing their sensitivity.
The two main types of camera sensors used are CCD (Charge Coupled Devices) or sCMOS (scientific Complementary Metal Oxide Semiconductors). Which camera you choose to use depends on the sample being imaged and the required frame-rate, field of view, resolution and sensitivity.
How the cameras work
All camera sensors are made up of an array of pixels which collect photons of light. Each pixel is composed of a photo-sensitive photodiode with silicon coupled to an electron storage well. When the photons hit the silicon substrate, photoelectrons are generated.
The electrons are stored in electron storage wells and then transferred (readout) to an amplifier. The amplifier reads the electrons and converts them to a voltage. Adjacent to the amplifier is an analog-digital (AD) converter which measures the voltage created by the electrons and translates this into a digital signal.
The more photons that hit the camera sensor, the more charge, and therefore signal, generated. The number of photons reaching the camera sensor is determined by the length of time exposed to light, the wavelength detected and the intensity of light. Each pixel in a CCD camera has a maximum number of electrons it can hold before it is saturated. This is known as the Full Well Capacity, and is dependent on the size of the pixel; the larger the pixel, the more electrons that can be collected before it is saturated.
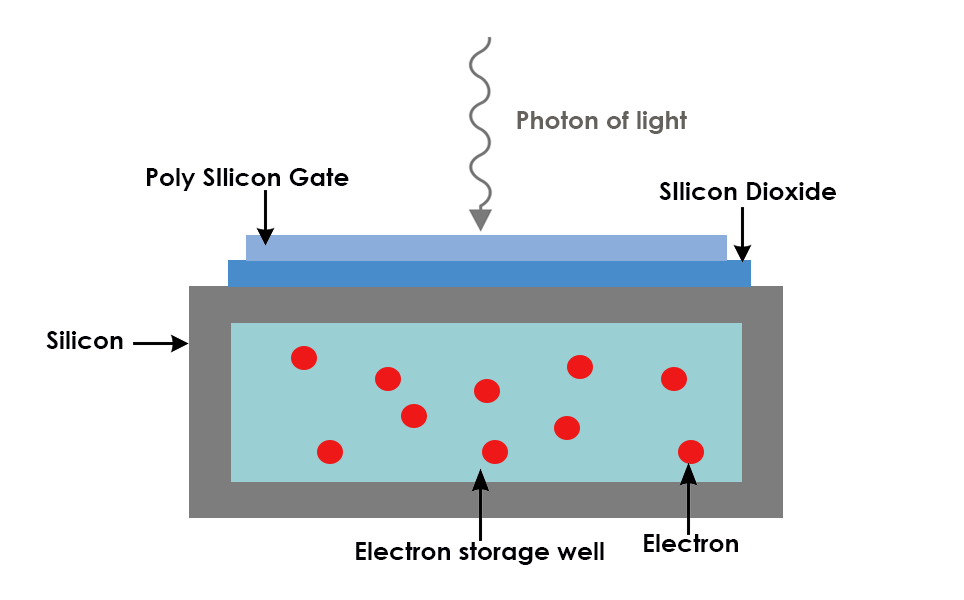
Sensitivity and signal-to-noise ratio
The sensitivity of a camera determines how clear the image produced is. A camera with high sensitivity has a high signal-to-noise ratio, as it has a high capacity to capture signal from the surrounding noise. To improve the signal-to-noise ratio, the signal can be increased and/or the noise can be decreased. A longer exposure time can also improve the signal-to-noise ratio.
Colour cameras usually have a lower resolution and sensitivity than monochrome cameras, therefore, unless multiple wavelengths are being imaged at the same time, monochrome cameras are best to use for fluorescence imaging.
Sensitivity is particularly important in low-light imaging, for example, low-light fluorescence, calcium imaging and single molecule imaging, or if small changes in fluorescence need to be detected.
Quantum Efficiency (QE)
The QE is the rate that sensors convert photons to electrons. This mainly matters in low-light imaging, as when there is lots of light, a small QE still means that plenty of light is detected and converted to electrons. The peak QE is the wavelength that the camera best converts photons to electrons.
Read noise
The noise generated by the camera electronics as the charge in the pixels is transferred to a digital signal. A lower read noise enables detection of weak signals.
Dark noise
Dark noise affects sensitivity and therefore image clarity. It is noise caused by the liberation of electrons in the chip by environmental heat. Dark noise can be reduced by cooling the camera, but this is often expensive and may cause vibrational interference to electrophysiology experiments.
Frame rate
Frame rate is the number of frames, or images, that are captured by the camera per second. Each camera has a maximum number of frames that it can image when using the whole camera chip. Frame rate is important in fast events such as calcium imaging, patch clamping and signalling events.
CMOS cameras usually have a higher frame rate than CCD cameras. How quickly the camera signal is converted from an analog to digital signal also affects the frame rate, for example, a 16 bit sensor can transmit more data faster than an 8bit sensor.
Frame rate can be improved by binning (see below), decreasing exposure time, reducing the region of interest being viewed and changing the PC connection.
A high frame rate, for example 5000 fps (frames per second), may not be utilised in some applications as there may not be enough light emitted from the sample to achieve the fast frame rate.
Binning
Binning is used to increase the frame rate of a camera sensor. Electrons from adjacent pixels are combined in the serial register and readout together. E.g. in 2x2 pixel binning, information from 4 adjacent pixels are combined and readout as one ‘super pixel’.
Binning increases the frame rate and signal-to-noise ratio but reduces spatial resolution.
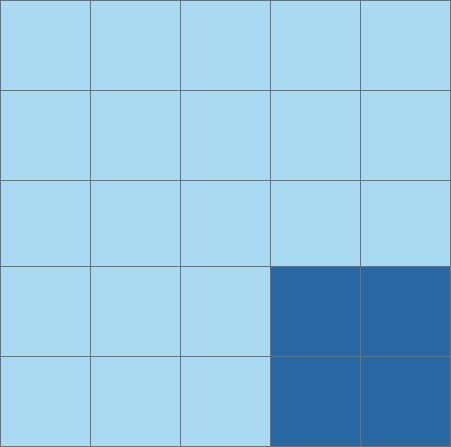
Resolution
This is the minimum point to point distance of the sample that can be distinguished as separate points on the image. Camera resolution depends on the number of pixels and the pixel size. A larger number of smaller pixels gives a higher resolution. Resolution is also affected by the numerical aperture (NA) of the objective.
Resolution is particularly important in morphological imaging, patch clamping and network studies.
CCD sensors
In CCD cameras, electrons in each pixel are passed along into the serial register. The serial register passes electrons one at a time to the amplifier and AD converter, which translates the voltage into a signal.
As every pixel is read in the same way, by the same AD converter, the AD converter has a lot of pixels to read quickly. The faster the frame rate of the camera, the greater the uncertainty at the AD converter, therefore the greater the read noise of the camera.
CCD cameras are ideal for fluorescence imaging. They produce black and white images, although, red, green or blue coloured filters can be placed on top of each pixel, allowing one primary colour to be read from each pixel.
As CCD cameras have been available for a number of years, there is a lot of choice in pixel size, colour and price.
Limitations of CCD cameras include a higher read noise as well as limited frame rates in order to reduce the read noise.
EMCCD sensors
EMCCD cameras are CCD cameras with an EM register. An EM register adds more electrons to the signal, amplifying it before it is read. This makes EMCCD cameras have a high speed and high sensitivity. However, there is an uncertainty as to how much the electrons have been multiplied. This is known as the X noise factor.
EMCCD cameras are ideal for low-light imaging, for example single molecule fluorescence, as these applications are limited by the read noise.
These cameras are usually a lot more expensive than regular CCD cameras.
sCMOS sensors
In sCMOS cameras, each pixel has its own amplifier, and one column of pixel connects to one AD converter. This results in sCMOS cameras having a faster frame rate compared to CCD cameras. They also have a lower read noise, as each AD converter has more time to read the electrons, so can read them more slowly than the one AD converter in a CCD camera.
sCMOS cameras have a large field of view and high resolution. They are used for high-end fluorescence imaging due to the fast frame rates, high dynamic range and low noise. In low-light environments, they can be used in conjunction with pixel binning to improve their photon collection. However, binning is less advantageous with sCMOS sensors than with CCD sensors.
Comparative table
| Hamamatsu Orca Flash 4.0 LT Plus | |||||
Application | Fluorescence microscopy, electrophysiology | Fluorescence microscopy, live cell imaging, confocal microscopy | Fluorescence microscopy | Fluorescence microscopy, dual colour imaging | Fluorescence microscopy | Fluorescence microscopy, electrophysiology, calcium imaging, confocal microscopy |
Sensor type
| CCD | sCMOS | sCMOS | sCMOS | sCMOS | sCMOS |
Peak QE | 75% at 600nm | 95% | 82% at 560nm | 82% at 560nm | 80% | 95% |
Pixel size | 6.45μm x 6.45μm | 11µm x 11µm | 6.5 μm × 6.5 μm | 6.5 μm × 6.5 μm | 6.5 μm × 6.5 μm | 6.5 μm × 6.5 μm |
Full Well Capacity (electrons) | >14,000 | 80,000 | 30,000
| 30,000 | 15,000 | 45,000e- (Combined Gain) 10,000e- (High Gain) |
Read Noise (RMS)
| <7 electrons with 50MHz readout | 1.6 electrons | 1.5 electrons | 1.6 electrons | 1.4 electrons (fast scan) 1.0 electron (standard scan) 0.7 electrons (ultra quiet scan) | 1.6 electrons 1.0 (quiet scan mode) |
Frame Rate (Frames per second) | 22 or 31 with 2x2 binning | 40 @ 16-bit 80 @ 12-bit | 30 | Up to 100 | Up to 100 | 43 |
Dark Current (electrons /pixel /second) | 0.036 at +15°C regulated | 0.55 (cooled to -20°C) | 0.6 (cooled to +10°C) | 0.06 (cooled to -10°C) 0.006 (cooled to -30° C) | 0.5 (-5°C) 0.2 (-15°C) | 0.5 (cooled to -20°C) |
Sensor Cooling | 0ºC stabilized at 23ºC ambient Thermoelectric cooling with convection | Forced air -10℃ | Passive air 10℃ | Forced air -10℃ | Forced air -5 ℃ Water -5 ℃ or -15 ℃ | Forced air -20°C Liquid -25°C Can switch between water and air cooling
|
Binning | 1x1, 2x2, 4x4, 6x6, 8x8, 12x12, 16x16 | 2x2 | 2x2, 4x4 | 2x2, 4x4 | 2x2 | 2x2 |
If you require further assistance, please do not hesitate to contact the team at Scientifica.
Find out about Scientifica's latest product releases, company news, and developments through a range of news articles, customer interviews and product demonstration videos.