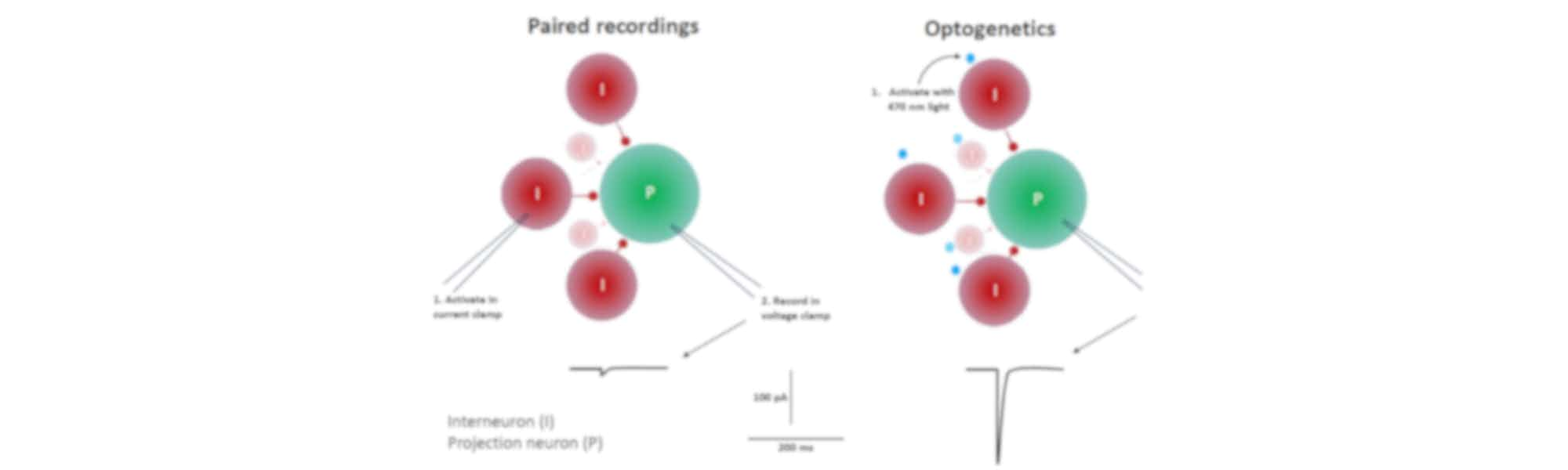
At the bench: Big changes in small circuits
Scientifica’s 'At the bench' articles are a series of posts written by researchers on their particular area of study and laboratory techniques.
The brain is composed of a complicated array of neuronal pathways between brain regions (macrocircuits) and also smaller circuits within brain regions (microcircuits) that ultimately coordinate in time and space to generate our equally complex ability to sense, integrate, think about and react to an ever-changing environment.
What sets us apart from the robots is our ability to adapt to these changes in the environment. Unlike computer circuits, the connections between and within our macro- and microcircuits, otherwise known as synapses, are capable of plastic changes, a process known as synaptic plasticity. It is this ability to shift the strength of synapses in short and long term fashions that underlie our ability to acquire and store information.
Historically, assessing plastic changes at synapses between macrocircuits in the brain has presented fewer technical challenges than microcircuit synapses. If one generates the brain slice at the right angle, one can often maintain the macrocircuit of interest and electrically activate the presynaptic inputs to interrogate the strength of specific inputs onto the postsynaptic cell of interest. However, this approach is not possible when assessing microcircuits.
The answer to this has been electrophysiologically recording from two or more neurons simultaneously (i.e. paired recordings), such that activating one neuron by injecting current activates that cell, which then releases neurotransmitter onto the postsynaptic cell from which you are recording with a second pipette. This approach is both annoyingly difficult for collecting the large amounts of data required to describe synaptic plasticity events fully and subject to limitations in the ability to detect changes in strength at synapses because the currents generated when one cell alone releases transmitter onto the other are quite weak. The result has been an accretion of data on synaptic plasticity at macrocircuits.
The problem is that microcircuits, despite their tiny name, can make a big difference for brain function. Microcircuits often form between sparsely populated interneurons and the abundant principal projection neurons of a brain nucleus. Despite the low numbers of interneurons across brain regions in general, they are capable of powerfully influencing projection neuron output either by releasing inhibitory neurotransmitters at key points onto a neuron or releasing modulatory neurotransmitters that are capable of shaping projection neuron output.
Now, optogenetics can be coupled with classical whole cell patch clamp electrophysiology to solve the technical challenges that have limited our ability to assess plastic changes at microcircuits. By expressing channelrhodopsin-2 (ChR2) in genetically identified interneurons of interest within a nucleus and recording from postsynaptic projection neurons, a simple flash of light is capable of eliciting an action potential from the population of interneurons. This provides a large postsynaptic current that can now be assessed for its ability to change in strength over time1,2.
This wholesale approach to assessing microcircuit plasticity now allows for rigorous characterization of microcircuit plasticity. This simple, yet elegant, approach of interfacing optical control with standard electrophysiological methods is transforming our ability to assess healthy and pathological synaptic changes at the teaming tiny circuits of the brain.
- Mathur B.N., Tanahira C., Tamamaki N., Lovinger D.M. Voltage drives diverse endocannabinoid signals to mediate striatal microcircuit-specific plasticity (2013) Nature Neuroscience doi: 10.1038/nn.3478
- Patton M.H., Roberts B.M., Lovinger D.M., Mathur B.N. Ethanol Disinhibits Dorsolateral Striatal Medium Spiny Neurons Through Activation of A Presynaptic Delta Opioid Receptor (2015) Neuropsychopharmacology doi: 10.1038/npp.2015.353
About the author:
Brian N. Mathur, PhD is an Assistant Professor at the University of Maryland School of Medicine in Baltimore, Maryland, USA. His lab is focused on the neural circuitry of action learning and control in normal and pathological states. For more information, see www.mathurlab.org.
Banner image credit: Brian Mathur