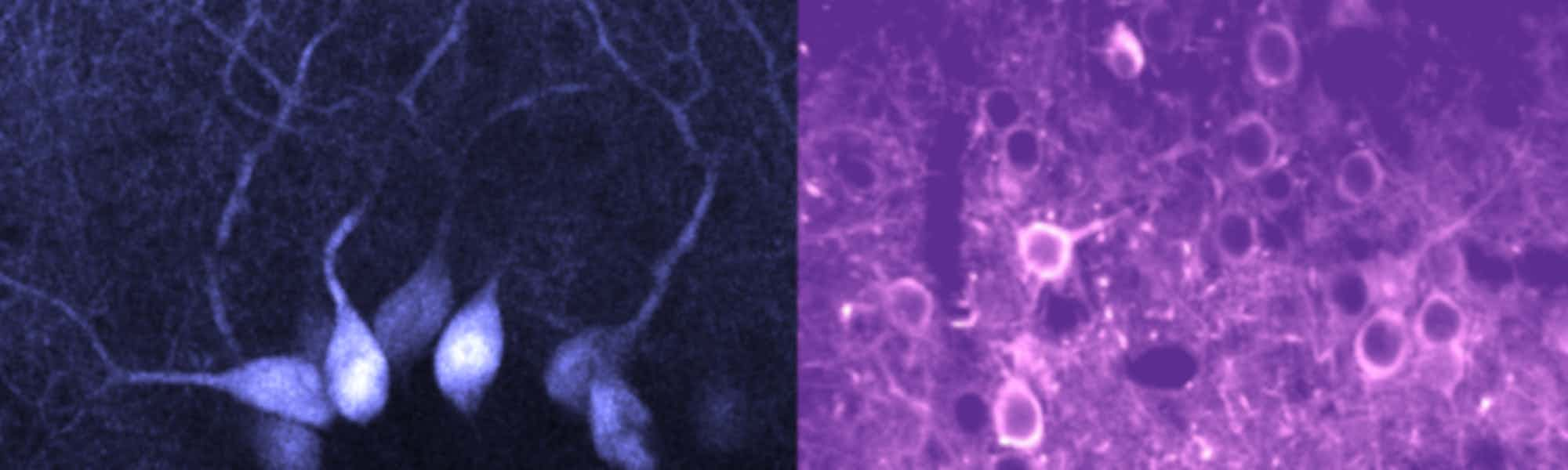
Navigation: More than a hippocampal affair
By Veronique van den Berghe
Professor Matteo Carandini, who runs a joint research group with Professor Kenneth Harris at University College London, kicked off the 2018 NEUReka! seminar series at the Centre for Developmental Neuroscience, King’s College London. During his talk he illustrated how brain areas outside the hippocampus, such as the primary visual cortex and the posterior parietal cortex, are also involved in navigation.
Navigation is strongly driven by vision. Imagine asking for directions to the station and you are told to take the second street on your left, turn right at the red house and continue straight on. Obviously, you would need to see these landmarks before deciding to make a turn in order to reach your destination. But how are vision, navigation and decision combined in the brain?
Prof. Carandini and his team demonstrated that spatial information is already present in the primary visual cortex (V1) of mice navigating a virtual environment (Saleem et al., 2017). Mice traversed a virtual corridor formed by two visually matching segments with alternating types of landmark - a grating and a plaid spaced 20 cm apart (Figure 1A-A’’). Calcium imaging during this task showed some V1 neurons with a symmetric response profile, however most neurons responded very differently to the visually similar stimuli. This asymmetric response was especially apparent when plotting maximum responses against position in the virtual corridor (Figure 1B). V1 neurons therefore seem to carry non-visual signals that are not explained by deviations in pupil position and diameter, running speed or reward.
To tease out whether these signals represent the brain’s navigational information, neuronal activity was simultaneously recorded in the V1 and the CA1 of the hippocampus. Similarly to the calcium imaging experiments, the majority of V1 neurons had asymmetric responses whereas CA1 place cells fired in a single position along the corridor. The animal’s subjective position was assessed with a reward (at least one lick in the reward zone resulted in a water reward, Figure 1C). Mice had to rely on vision to perform the task correctly and made more errors when visual contrast was reduced. The subjective position along the corridor, decoded from firing of V1 and CA1 neurons, correlated well for both these areas with the actual position during correct trials (Figure 1D,D’). On the other hand, incorrect trials discriminated between the animal’s subjective and actual positions. Interestingly, V1 and CA1 decoding mistakes correlated with over- and under- estimation of the position in the corridor in early and late trials, respectively (Figure 1E-F’, deviation above and below diagonal). Spatial signals related to the mouse’s subjective position thus already appear in sensory systems such as the primary visual cortex.
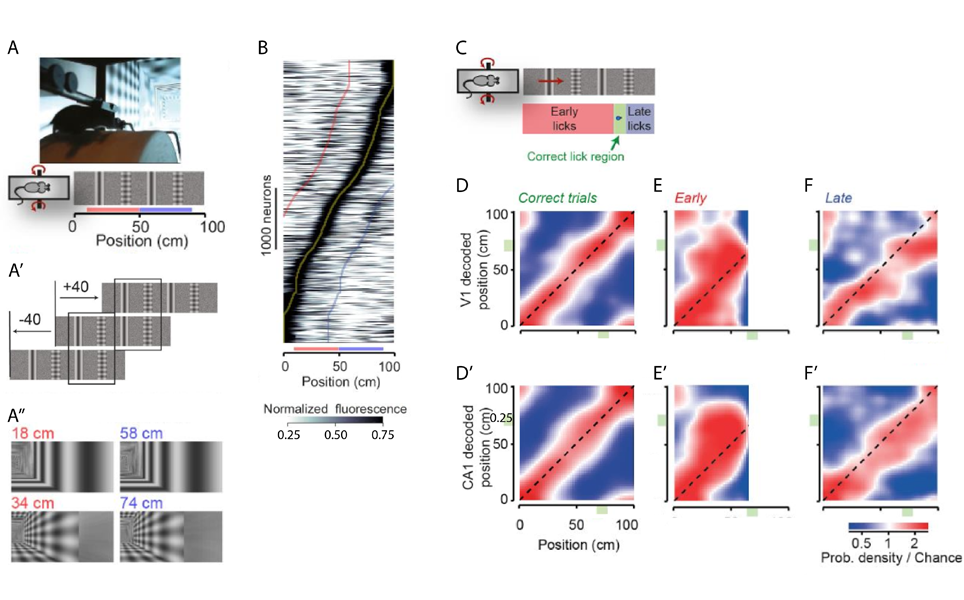
A, A’ Mice ran on a cylindrical treadmill to navigate a virtual corridor with two landmarks (a grating and a plaid). Repetition of the landmarks created two visually identical segments (red and blue bars). A’’ Screen images for the right visual field indicating the similarity of positions 40 cm apart.
B Normalised response of V1 neurons as a function of distance in the virtual corridor ordered by the position of their maximum response (yellow line). Red and blue lines indicate response to the visually similar position 40 cm before or after the maximum response, respectively.
C When mice licked in a reward zone (green area) they received a water reward. Trials with too many licks before the reward zone were terminated and labelled ‘early trials’, whereas in ‘late trials’ the mouse only licked after the reward zone.
D, D’ Distribution of the position decoded from simultaneously recorded V1 (D, y-axis) and CA1 (D’, y-axis) neurons as a function of the animal’s actual position (x-axis).
E- F’ Density maps like in D and D’ when the animal licked early (E, E’) or late (F, F’).
Modified from Saleem et al., 2017.
In rodents the posterior parietal cortex (PPC) encodes combinations of spatial attributes, such as position and body movement. In humans and primates this area is much bigger and is thought to have a role in decision-making during movement based on vision. In the second part of his talk, Prof. Carandini described a different virtual environment where mice used vision to perform a T-maze task (Krumin et al., 2017). Vertical grating on one side of the corridor indicated the arm where mice would receive a water reward, whereas the choice of the other arm resulted in a brief white noise sound (Figure 2A,A’). Mice would already head towards the intended arm before the end of the corridor, and they would do so earlier when grating contrast increased. Calcium imaging demonstrated that PPC neurons were active at a specific position in the corridor. Initially neurons were active in both leftward and rightward trials, but as mice progressed in the corridor PPC neurons separated into cells preferring leftward or rightward trials (Figure 2B). A clear response field or “position-heading field” was identified when the density of a neuron’s calcium activity was plotted in function of position and heading (Figure 2C). Together, position in the corridor and heading direction were sufficient to predict activity of PPC neurons, and the position-heading model was not improved by taking into account the animal’s decision. Unlike in humans and primates, when mice use vision to guide navigation, the parietal cortex encodes position and heading rather than decisions to turn left or right.
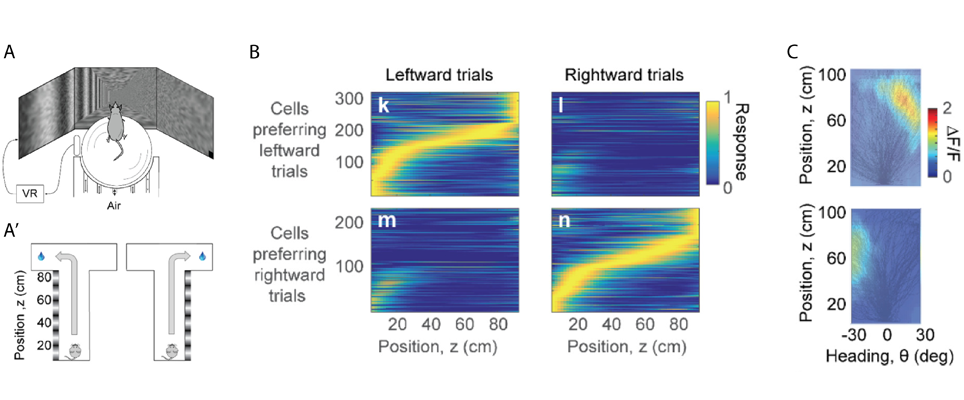
A, A’ Mice navigated a virtual T-maze on an air-suspended ball. The animals received a water reward for choosing the correct arm while hearing a brief white noise for the wrong arm. Gratings on one side of the corridor indicated which arm to choose to receive the reward.
B Response of PPC neurons in function of distance in the virtual corridor. Cells were active during a specific position and the majority of activity was selective to trial outcome. C Two neurons with their position-heading fields. Black lines show the trajectories taken by the animal in different trials during a behavioural session. Both neurons were active towards the end of the corridor while the mouse was heading to the right (upper panel) or left (lower panel).
Modified from Krumin et al., 2017.
In 2014, John O’Keefe, May-Britt and Edvard Moser received the Nobel Prize in Physiology or Medicine for their discoveries of hippocampal place cells and entorhinal grid cells. The committee described their work as “a comprehensive positioning system, an inner GPS, in the brain”. The interesting data presented by Prof. Carandini indicate that there is still a lot to discover and that integrated complex spatial information is already present in areas upstream of the hippocampus such as the visual and parietal cortex.
References
Krumin, M., Harris, K.D., and Carandini, M. (2017). Decision and navigation in mouse parietal cortex. BioRxiv.
Saleem, A.B., Diamanti, E.M., Fournier, J., Harris, K.D., and Carandini, M. (2017). Coherent representations of subjective spatial position in primary visual cortex and hippocampus. BioRxiv.
Scientifica supports the NEUReka! Seminar Series to help them book speakers from top research institutions around the world.
Find out about Scientifica's latest product releases, company news, and developments through a range of news articles, customer interviews and product demonstration videos.